Traumatic brain injury (TBI) is a universal health concern as it is has been identified as a “silent epidemic” in civilians and the “signature injury” in U.S. service members (Soldiers) in the Iraq and Afghanistan wars1,2. Each year in America, 2.53 million civilians visit the emergency department after suffering a TBI, of which 56,800 die and over 288,000 require hospitalization and long term supportive care3. In addition, 400,000 TBI diagnoses have been confirmed in the Operation Enduring Freedom and Operation Iraqi Freedom conflicts4. 80% of these Soldiers will experience comorbid psychiatric diagnoses and are >1.5x more likely to die from suicide than Soldiers without TBI5,6. These statistics correspond to civilian research where TBI has also been linked to suicide as well as mood and anxiety disorders7,8. Collectively, these consequences have major repercussions for TBI patients’ families with psychological, emotional, and financial effects.
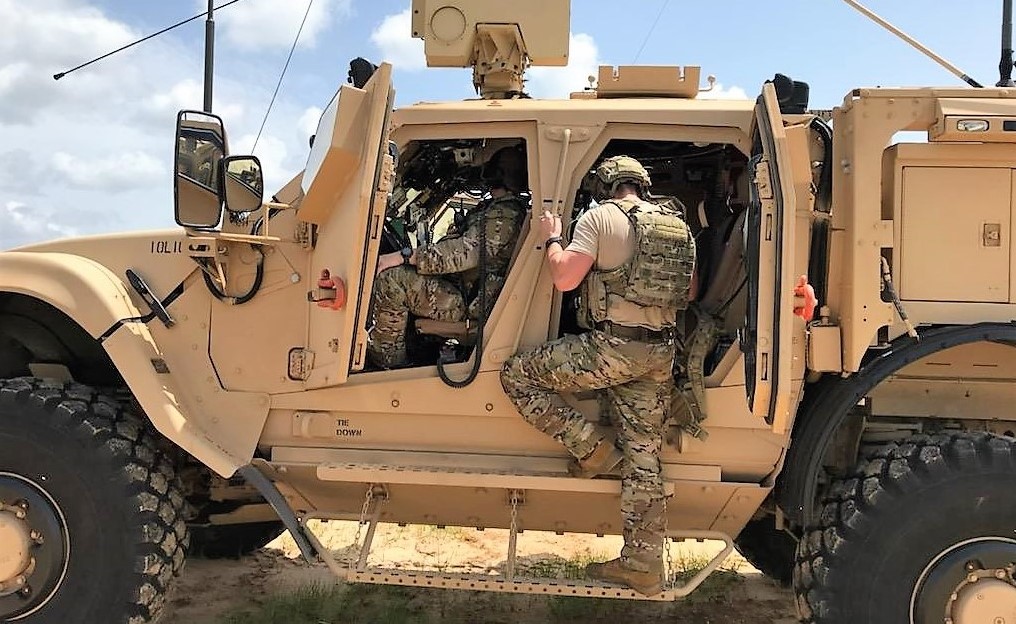
Presently, there are no neuroprotective or regenerative Food and Drug Administration (FDA)-approved TBI treatments. TBI pathologies including brain swelling and intracerebral hemorrhage are often treated with hyperosmolar fluids, decompressive craniectomy, and surgical evacuation, all of which are associated with multiple risk factors9. Consequently, a safe and neuroprotective TBI treatment is sorely needed. Preclinical evaluation of novel therapies in our TBI pig model10-13 is likely more predictive of human responses and outcomes due to the anatomical similarities between humans and pigs including brain size, gyrencephalic cytoarchitecture, and high white-to-gray matter ratios. These characteristics should be considered when evaluating the efficacy of novel therapeutics as they have a significant impact on TBI pathologies (e.g. cell death, excitotoxicity, inflammatory responses, intracerebral hemorrhage, and edema) and recovery mechanisms14,15.
- Brain size is critically important in modeling TBI as smaller rodent brains can tolerate much greater angular acceleration forces than animals with larger brains as shearing forces and inertial loading are directly related to brain mass16.
- Humans and pigs also have gyrencephalic brains, while rodents have lissencephalic brains. Gyrification significantly influences the movement of the brain within the cranium during TBI, as well as the maximum mechanical stress applied to neural tissues16.
- Human and pig brains are also composed of large white matter volumes (>60%), compared to rodents (<12%)17,18. White matter composition is important when modeling TBI as white matter is more susceptible than gray matter as it possesses 3-5x less microvasculature and limited collateralization19-21.
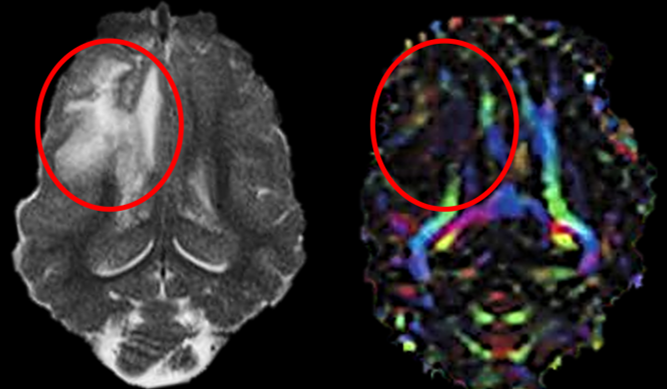
We believe our pig TBI model could serve as a translational platform for studying TBI sequelae across injury severities and identifying novel therapeutics.
- 1Meaney, D.F., B. Morrison, and C. Dale Bass, The mechanics of traumatic brain injury: a review of what we know and what we need to know for reducing its societal burden. J Biomech Eng, 2014. 136(2): p. 021008.
- 2Taylor, B.C., et al., Prevalence and costs of co-occurring traumatic brain injury with and without psychiatric disturbance and pain among Afghanistan and Iraq War Veteran V.A. users. Med Care, 2012. 50(4): p. 342-6.
- 3Centers for Disease Control and Prevention (2019). Surveillance Report of Traumatic Brain Injury-related Emergency Department Visits, Hospitalizations, and Deaths—United States, 2014. Centers for Disease Control and Prevention, U.S. Department of Health and Human Services.
- 4Chase, R.P. and R.L. Nevin, Population estimates of undocumented incident traumatic brain injuries among combat-deployed US military personnel. J Head Trauma Rehabil, 2015. 30(1): p. E57-64.
- 5Carlson, K.F., et al., Psychiatric diagnoses among Iraq and Afghanistan war veterans screened for deployment-related traumatic brain injury. J Trauma Stress, 2010. 23(1): p. 17-24.
- 6Brenner, L.A., R.V. Ignacio, and F.C. Blow, Suicide and traumatic brain injury among individuals seeking Veterans Health Administration services. J Head Trauma Rehabil, 2011. 26(4): p. 257-64.
- 7Scholten, A.C., et al., Prevalence of and Risk Factors for Anxiety and Depressive Disorders after Traumatic Brain Injury: A Systematic Review. J Neurotrauma, 2016. 33(22): p. 1969-1994.
- 8Silver, J.M., et al., The association between head injuries and psychiatric disorders: findings from the New Haven NIMH Epidemiologic Catchment Area Study. Brain Inj, 2001. 15(11): p. 935-45.
- 9Xiong, Y., A. Mahmood, and M. Chopp, Current understanding of neuroinflammation after traumatic brain injury and cell-based therapeutic opportunities. Chin J Traumatol, 2018. 21(3): p. 137-151. 46.
- 10Baker, E.W., et al., Scaled traumatic brain injury results in unique metabolomic signatures between gray matter, white matter, and serum in a piglet model. PLoS One, 2018. 13(10): p. e0206481.
- 11Kinder, H.A., et al., Traumatic Brain Injury Results in Dynamic Brain Structure Changes Leading to Acute and Chronic Motor Function Deficits in a Pediatric Piglet Model. J Neurotrauma, 2019. 36(20): p. 2930-2942.
- 12Baker, E.W., et al., Controlled Cortical Impact Severity Results in Graded Cellular, Tissue, and Functional Responses in a Piglet Traumatic Brain Injury Model. J Neurotrauma, 2019. 36(1): p. 61-73.
- 13Kinder, H.A., et al., Controlled Cortical Impact Leads to Cognitive and Motor Function Deficits that Correspond to Cellular Pathology in a Piglet Traumatic Brain Injury Model. J Neurotrauma, 2019. 36(19): p. 2810-2826.
- 14Baltan, S., et al., White matter vulnerability to ischemic injury increases with age because of enhanced excitotoxicity. J Neurosci, 2008. 28(6): p. 1479-89.
- 15Jordan, L.C., J.T. Kleinman, and A.E. Hillis, Intracerebral hemorrhage volume predicts poor neurologic outcome in children. Stroke, 2009. 40(5): p. 1666-71.
- 16Vink, R., Large animal models of traumatic brain injury. J Neurosci Res, 2018. 96(4): p. 527-535.
- 17Tanaka, S.C., B.W. Balleine, and J.P. O’Doherty, Calculating consequences: brain systems that encode the causal effects of actions. J Neurosci, 2008. 28(26): p. 6750-5.
- 18Nakamura, M., et al., Experimental investigation of encephalomyosynangiosis using gyrencephalic brain of the miniature pig: histopathological evaluation of dynamic reconstruction of vessels for functional anastomosis. Laboratory investigation. J Neurosurg Pediatr, 2009. 3(6): p. 488-95.
- 19Borowsky, I.W. and R.C. Collins, Metabolic anatomy of brain: a comparison of regional capillary density, glucose metabolism, and enzyme activities. J Comp Neurol, 1989. 288(3): p. 401-13.
- 20Nonaka, H., et al., The microvasculature of the cerebral white matter: arteries of the subcortical white matter. J Neuropathol Exp Neurol, 2003. 62(2): p. 154-61.
- 21Peters, A. and C. Sethares, Oligodendrocytes, their progenitors and other neuroglial cells in the aging primate cerebral cortex. Cereb Cortex, 2004. 14(9): p. 995-1007.