The TNRR Laboratory has assembled a diverse team of experts to assess the capabilities of novel stem cell therapies for the treatment of acute and chronic diseases and injuries. This cross disciplinary effort enables an in depth study of human stem cell differentiation and functionality in disease models, thus providing valuable insight into how these cells may ultimately function in patients. Our laboratory also studies stem cells from livestock sources to assess potential utility in agricultural, veterinary, and human research models.
Embryonic stem cells (ESCs) are stem cells derived from the inner cell mass of developing embryos. These cells are pluripotent and therefore can turn into any cell type in the body. This quality makes ESCs a good candidate for cell therapy.
Induced pluripotent stem cells (iPSCs) are a novel class of stem cells that can also turn into any cell type in the body. However, what makes these cells unique is they can be derived by overexpressing a combination of six reprogramming genes, thus eliminating the need for isolating cells (i.e. ESCs) from developing embryos. In fact. recent research has shown that iPSCs can be generated from a patient’s own skin cells. Patient iPSCs have similar plasticity to ESCs and are able to differentiate into neural stem cells (iNSCs) which can then be used to effectively treat ischemic stroke and other neural injuries in animals models1,2.
In 2010, the TNRR Laboratory was the first to utilize porcine iPSCs to generate chimeric animals3. At the time, this had not been accomplished with ESCs or iPSCs in any species outside of rodents. Our work has opened the doors for an in‐depth study of iPSC tumorigenicity, autologous transplantation, and other key aspects that are necessary to move iPSC therapies to clinical trials, while ensuring safety and efficacy.
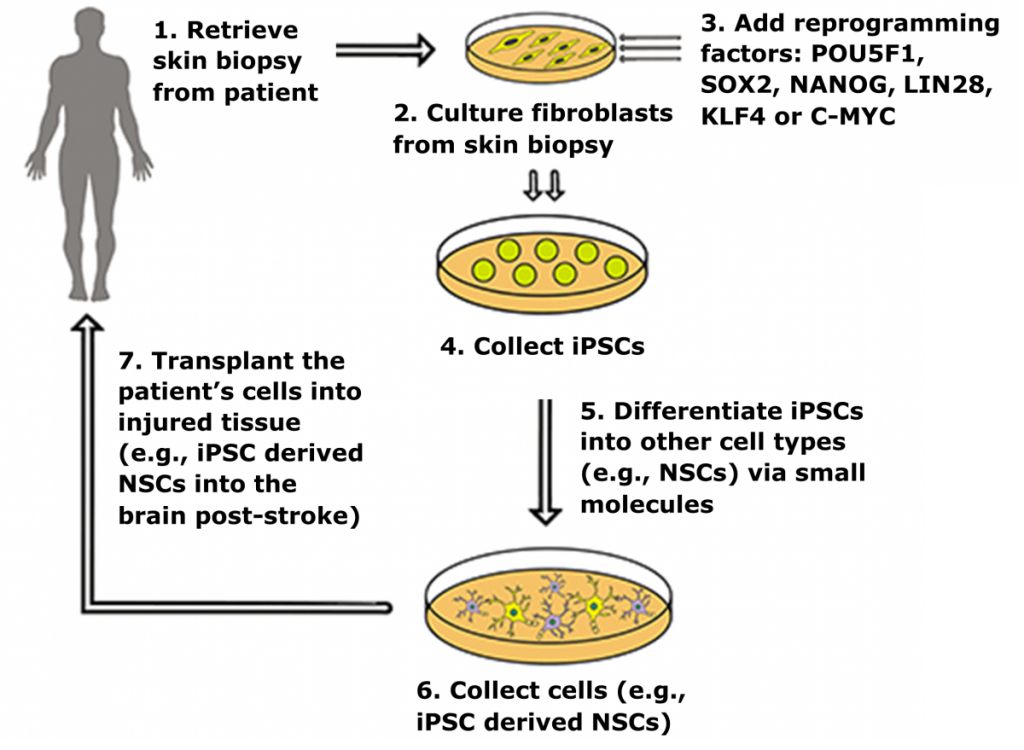
iPSC derived neural stem cells (iNSCs) have a dual mode of therapeutic action by 1) serving as a cell replacement therapy by differentiating into neurons and glia that integrate into surrounding tissues and 2) producing neuroprotective and regenerative growth factors that stabilize and promote healing of damaged parenchyma4-6. In rodent stroke models, transplanted iNSCs reduce microglia activation, glial scar formation, promote angiogenesis, reduce tissue atrophy, and differentiate to mature neuronal cell types that engraft into the host brain7,8.
In our pig stroke model, we reported for the first time that intraparenchymal transplantation of human iNSCs improved tissue recovery. Longitudinal MRI also provided evidence of recovery in brain metabolism, white matter integrity, and cerebral blood perfusion post-iNSC transplantation. Histologic assessment demonstrated iNSCs resulted in neural protection, decreased microglial activation, and stimulated endogenous neurogenesis. Transplanted iNSCs survived for 12 weeks in the pig brain and differentiated into neuronal and glial cells.
We also demonstrated for the first time that iNSCs improved functional recovery post-stroke, with appetite and body posture exhibiting the most improvement in the iNSC-treated group9. These results demonstrated that iNSCs have significant potential as a regenerative and cell replacement therapy in a robust translational large animal model, thus providing further insight into the potential of iNSCs as a therapeutic for patients.
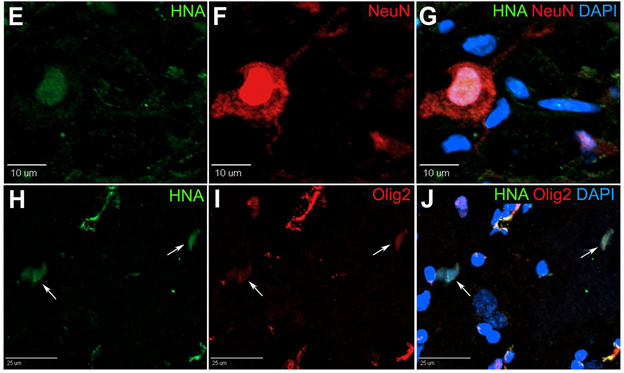
iPSCs specific to Leigh’s syndrome have also been generated by the TNRR Laboratory by reprogramming fibroblasts10. Leigh’s syndrome, a fatal neurodegenerative mitochondrial disorder in infants, is not fully understood and requires the generation of relevant patient- and disease-specific stem cell models. As such, we focused on the ability of non-viral and integration-free reprogramming methods to generate clinical-grade iPSCs. For the first time, we demonstrated derivation and characterization of a stable non-viral human iPSC line reprogrammed from a Leigh’s syndrome patient’s fibroblasts carrying a high abundance of mutant mitochondrial DNA. These unprecedented outcomes help us to further understand disease origins and develop personalized therapies for patients suffering from mitochondrial diseases.
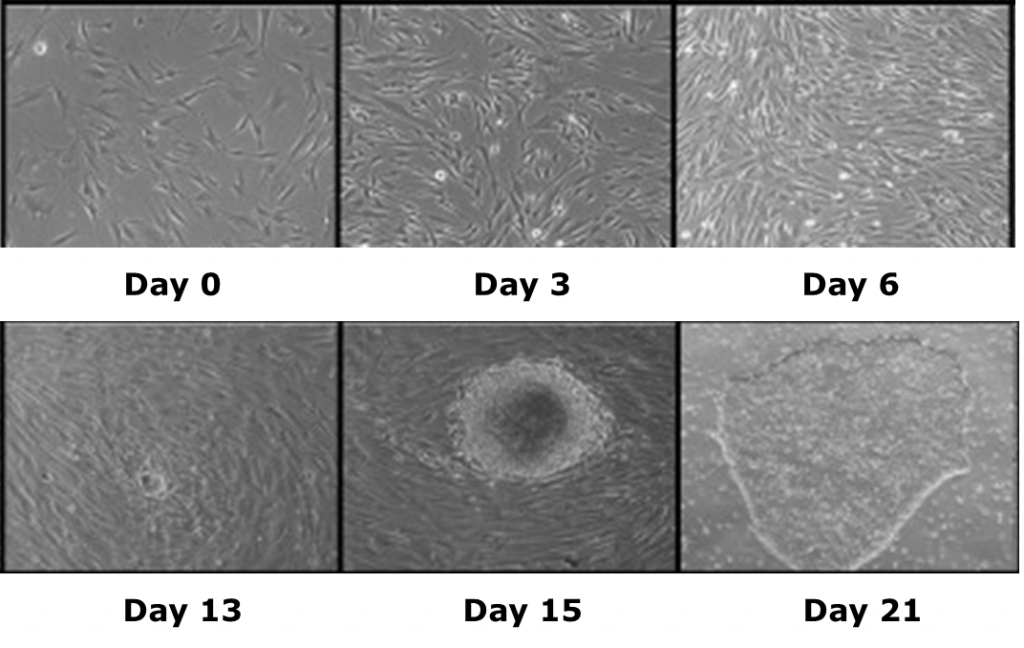
- 1Takahashi, K. & Yamanaka, S. et al., Induction of pluripotent stem cells from mouse embryonic and adult fibroblast cultures by defined factors. Cell, 2006. 126, p: 663–676.
- 2Baker, E.W., et al., Induced Pluripotent Stem Cell-Derived Neural Stem Cell Therapy Enhances Recovery in an Ischemic Stroke Pig Model. Sci Rep, 2017. 7(1): p. 10075.
- 3West, F., et al., Porcine induced pluripotent stem cells produce chimeric offspring. Stem cells and development, 2010. 19(8): p. 1211–1220.
- 4Oki, K., et al., Human-induced pluripotent stem cells form functional neurons and improve recovery after grafting in stroke-damaged brain. Stem Cells, 2012. 30(6): p. 1120-33.
- 5Polentes, J., et al., Human induced pluripotent stem cells improve stroke outcome and reduce secondary degeneration in the recipient brain. Cell Transplant, 2012. 21(12): p. 2587-602.
- 6Tornero, D., et al., Human induced pluripotent stem cell-derived cortical neurons integrate in stroke-injured cortex and improve functional recovery. Brain: a journal of neurology, 2013. 136: p. 3561–3577.
- 7Chang, D. J. et al. Contralaterally transplanted human embryonic stem cell-derived neural precursor cells (ENStem-A) migrate and improve brain functions in stroke-damaged rats. Experimental & molecular medicine, 2013. 45: p. e53.
- 8Chang, D. J., et al., Therapeutic potential of human induced pluripotent stem cells in experimental stroke. Cell transplantation, 2013. 22: p. 1427–1440.
- 9Lau, V. W., et. al., Human iNPC therapy leads to improvement in functional neurologic outcomes in a pig ischemic stroke model. Brain and behavior, 2018. 8(5): p. e00972.
- 10Grace, H. E., et. al., mRNA Reprogramming of T8993G Leigh’s Syndrome Fibroblast Cells to Create Induced Pluripotent Stem Cell Models for Mitochondrial Disorders. Stem cells and development, 2019. 28(13): p. 846–859.